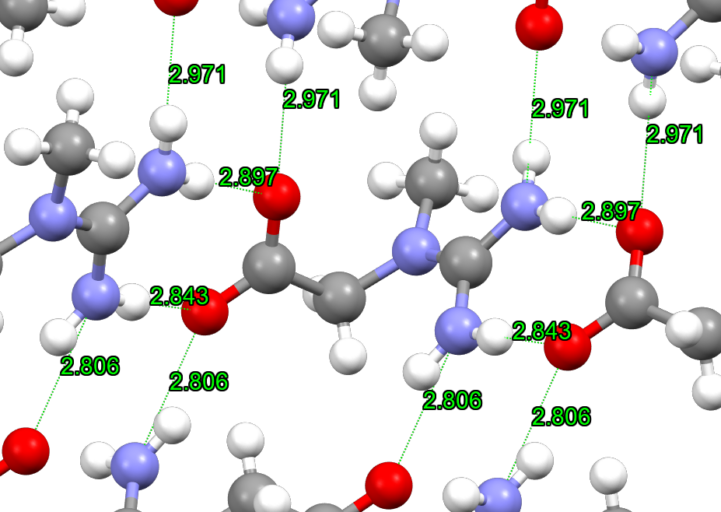
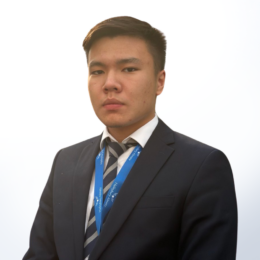
Author Background: Alikhan Serikuly grew up in Kazakhstan and currently attends Haileybury Almaty in Almaty, Kazakhstan. His Pioneer research concentration was in the field of chemistry and was titled “Chemistry of Foods”.
The synthesis of creatine takes place naturally in organs such as the liver, kidney, and pancreas, and creatine’s primary storage location is within skeletal muscle. It can be obtained through the consumption of animal-based foods or synthesized naturally within the human body. It plays a vital role in the energy metabolism of most vertebrates by capturing and storing high-energy phosphate groups and donating them to adenosine diphosphate (ADP) to restore depleted adenosine triphosphate (ATP) levels. This reversible process is facilitated by creatine kinase (CK). Following their initial introduction to the general public in the 1990s, creatine supplements have gained significant popularity and are predominantly utilized by athletes as an ergogenic aid (Close et al., 2o16). However, whole-body creatine deficiency most strongly affects the nervous system, with the potential to cause cognitive dysfunction, seizures, and behavioral impairments (Mercimek-Andrews & Salomons, 2009). Emerging research has provided evidence of additional positive effects of creatine supplementation on the health of non-athletes, thereby suggesting its potential use for the general public. The purpose of this research paper is to provide an in-depth exploration of the biochemical properties of creatine and its potential as a dietary supplement for the general public. This paper aims to investigate the synthesis, chemical properties, metabolism, and transportation of creatine, as well as its effects on sports performance, cognitive function, and potential applications in cancer treatment and antiviral activity. By reviewing existing literature and incorporating recent research findings, this paper aims to provide a comprehensive understanding of creatine and its potential benefits for individuals seeking to enhance physical performance, cognitive abilities, and overall health.
Figure 1. Creatine 3D structure (PubChem)
Creatine is a non-proteinogenic amino acid and a glycine derivative with the chemical formula C4H9N3O2. At room temperature, creatine is slightly soluble in water (13 g/L). Like all amino acids, it contains carboxyl and amino functional groups. Due to charge separation and the presence of O-H and N-H bonds, creatine can form hydrogen bonds that play an essential role in protein binding (Colas et al., 2020). The crystal structure of creatine contains hydrogen bonds between guanidine and carboxyl groups as well as charge attraction interactions. The lone pairs on all three nitrogen atoms are delocalized, explaining the trigonal planar geometry on the nitrogen atoms and equal lengths of C-N bonds of the guanidine group. The zwitterionic form is highly stabilized by resonance and is the major species at physiological pH (7.3), with pKa values of amino and carboxylic acid moieties estimated to be 12.7 and 3.8, respectively (PubChem). Owing to charge separation, creatine is a relatively reactive molecule.
Figure 2. Creatine bond angles and lengths (Arlin et al., 2014) in Mercury (Macrae et al., 2020)
Figure 3. Creatine crystal structure (Arlin et al., 2014) in Mercury (Macrae et al., 2020)
Creatine is naturally synthesized in the kidney and liver through the conversion of glycine and arginine, facilitated by the enzyme arginine:glycine amidinotransferase (AGAT). Guanidinoacetate (GAA), formed from the reaction between glycine and arginine, is then methylated by guanidinoacetate N-methyltransferase (GAMT), using S-adenosyl methionine (SAM) as the methyl donor. The result is creatine. Creatine’s cyclic form, creatinine, exists in equilibrium with its tautomer and creatine itself (Joncquel-Chevalier Curt et al., 2015).
Figure 4. Creatine biosynthesis pathway
Figure 5. Creatinine tautomerization
Creatine is transported through the bloodstream and taken up into the cell by the transmembrane protein creatine transporter, CreaT. Malfunctions or mutations of CreaT can result in creatine deficiency within the brain. Such a deficiency has the potential to cause extreme neurological disorders such as epilepsy and intellectual disability (Passi et al., 2022). CreaT belongs to the solute carrier transporter 6 family (SLC6), specifically the subgroup of GABA transporters (GATs). These transporters, including CreaT, are considered symporters as they transport Na+ ions along with their respective substrates in the same direction, utilizing the electrical potential gradient across the cellular membrane.
Colas and fellow researchers created homology models of CreaT based on the structurally similar serotonin transporter (hSERT) that shed light on the structural factors characterizing ligand binding to the active site of CreaT. One discovered feature is that transmembrane helix 10 (TM10) has a π helix that provides a specific conformation to the binding site and is believed to influence the substrate selectivity among proteins in the SLC6 family. Another feature is the deprotonated cysteine (C144), located on TM3, that is specific to CreaT. This cysteine plays an essential role in CreaT ligand binding because it allows for S-H-N hydrogen bonding with the guanidine group of the substrate. Complexes of CreaT with different ligands were studied, and it was revealed that optimal binding occurs when the distance between carboxylate and guanidine groups is approximately 4.5 – 5 Å, which corresponds to a linker with 2-3 carbon atoms (Colas et al., 2020).
Figure 6. Creatine binding to CreaT (Colas et al., 2020)
5. Metabolic Role.
About two-thirds of the total creatine in the body is stored in the form of phosphocreatine (PCr). PCr is created by creatine phosphorylation catalyzed by CK and serves as an energy buffer in skeletal muscles and the brain by supplying high-energy phosphates to convert ADP to ATP when needed. The enzymatic breakdown of PCr into Cr and inorganic phosphate (Pi) provides energy that is used to synthesize ATP from ADP and Pi.
Maintaining adequate ATP availability is crucial in situations where ATP levels have been depleted due to high energy demands. The capacity to restore depleted ATP levels assumes significance in sustaining optimal ATP availability. Approximately 95% of creatine is stored in skeletal muscle, with the remaining 5% distributed among other tissues, notably the brain (Balsom et al., 1994). The breakdown of creatine into creatinine occurs at a rate of approximately 1-2% per day. The degradation process is more pronounced in more physically active individuals, as well as individuals exhibiting higher lean muscle mass (Hultman et al., 1996). As a result, a person of average size may require a daily intake of 2-3 grams of creatine to maintain normal levels in their muscles. This requirement can vary depending on factors such as diet, muscle mass, and physical activity levels. Creatine stores in individuals following either a normal omnivorous diet or a vegan diet are usually not saturated. Therefore, to promote overall health, the recommended daily dietary intake of creatine may range from 2 to 4 grams per person (R. B. Kreider et al., 2017; R. B. Kreider & Stout, 2021).
Figure 7. Phosphocreatine structure
Improved exercise performance associated with creatine supplementation is caused by an increase in muscle creatine and PCr levels (Buford et al., 2007). Studies have shown that individuals who take creatine experience a performance increase of approximately 10-20% in various high-intensity activities, including fitness and weight training (Izquierdo et al., 2002; R. Kreider et al., 1998; Wiroth et al., 2001). These benefits have been observed in both men and women across different age groups. Consequently, it is widely acknowledged among scientists that creatine supplementation is an important and valuable ergogenic aid for athletes. The quickest and most effective way to elevate muscle creatine levels is to consume 5 g of creatine four times a day for 5-7 days (R. B. Kreider & Stout, 2021).
The brain is an organ with a high energy demand, which implies that creatine may offer potential cognitive benefits. Indeed, creatine supplementation boosts brain bioenergetics and reduces mental fatigue (Braissant et al., 2011). It has also been observed to enhance extended cognitive abilities in healthy individuals (Van Cutsem et al., 2020) and alleviate the decline in skill execution that arises from lack of sleep (Cook et al., 2011).
Creatine plays a complex role in the development and progression of cancer, because its benefits can be used by both tumor cells and healthy cells. It has demonstrated potential anti-cancer effects, acting as a power source for T cell activities (Campos-Ferraz et al., 2016; Di Biase et al., 2019) and similar effects have been observed with cyclocreatine (Miller et al., 1993). However, recent studies have unveiled the protumor effects of creatine, specifically in promoting cancer metastasis (Papalazarou et al., 2020; Zhang et al., 2021). Additionally, elevated levels of CK have been observed in certain cancers, such as lung cancer (Gazdar & et al., 1981) and prostate carcinoma (Feld & Witte, 1977). Inhibition of the SLC6A8 gene, responsible for creatine transport, has been shown to reduce PCr and ATP levels in cells and induce tumor apoptosis (Kurth et al., 2021). Despite ongoing research, the complete understanding of creatine’s role in cancer remains elusive. Therefore, caution is advised when considering creatine supplementation in the context of cancer.
Calculations were performed using (1) the Main Protease crystal structure coordinates as obtained from the Protein Data Bank (PDB) with PDBID: 6Y84 (Owen et al., 2023) and (2) the creatine crystal structure atomic coordinates downloaded from the Cambridge crystallographic database (CSD) CSD code: JOHJIB01 (Arlin et al., 2014). We used software from Biovia (Dassault Systèmes, San Diego, CA, USA). Docking studies were performed with the CDOCKER package in Discovery Studio 2020 version (Wu et al., 2003). Standard dynamics cascade protocol in Discovery Studio allows optimization of atomic coordinates and was also applied to the selected poses.
Figure 8. Creatine binding to SARS-CoV-2 main protease active site amino acids
This computational analysis uncovers the potential of creatine to intricately bind to the active site of SARS-CoV-2 main protease, suggesting its capacity as an inhibitor of this protease, which is known to play an essential role in viral replication by cleaving polyproteins to form multiple functional proteins, including those responsible for RNA replication (Qiao et al., 2021). Additionally, creatine has the potential to prevent the protease from dimerizing, which is necessary for its function. Despite the study’s reliance on computational analysis, these findings pave the way for the exploration of creatine as a promising candidate for effectively targeting and inhibiting the protease and therefore the activity of the virus.
Research indicates a potential exacerbation of liver damage caused by ethanol when taken together with creatine (Marinello et al., 2019). Despite previous suppositions, research has shown that neither anhydrous caffeine nor coffee hinders the performance boost from creatine, but simultaneous ingestion may cause digestive discomfort (Trexler et al., 2016).
Creatine monohydrate (CM) supplementation has been associated with anecdotal claims of side effects such as cramping or fluid balance disruption, but scientific research suggests that these concerns are largely unfounded (Dalbo et al., 2008; Lopez et al., 2009). In fact, Greenwood and others discovered that football players who supplemented with creatine experienced significantly less cramping, dehydration, muscle tightness and strains during training (Greenwood et al., 2003). Creatine has also been speculated to have a negative effect on kidney function. Certain fears about creatine and renal function stemmed from a case study involving a man with pre-existing kidney disease (Koshy et al., 1999; Pritchard & Kalra, 1998). Some concerns have centered around increased serum creatinine levels, which are often used as an indicator of kidney health. However, large-sample studies conducted over several years have not found any significant side effects, including adverse effects on renal function, resulting from CM supplementation in healthy athletes (Groeneveld et al., 2005; R. B. Kreider et al., 2003; Lugaresi et al., 2013; Poortmans & Francaux, 1999). To conclude, current evidence suggests that CM supplementation is safe within recommended guidelines.
In conclusion, creatine supplementation offers various benefits in terms of sports performance enhancement, cognitive function improvement, and potential anticancer properties without any known adverse side effects. Furthermore, our computational study reveals creatine’s potential as an antiviral agent that arises from its ability to bind to the SARS-CoV-2 main protease. Creatine’s wide availability and relatively low cost make it an intriguing research target to investigate as a SARS-CoV-2 treatment. While further research is needed to explore its full range of applications and mechanisms of action, the current evidence supports the use of creatine as a valuable nutritional supplement for both athletes and the general population.
Arlin, Jean-Baptiste, Ritu M. Bhardwaj, Andrea Johnston, Gary J. Miller, Julie Bardin, Fraser MacDougall, Philomena Fernandes, Kenneth Shankland, William I. F. David, and Alastair J. Florence. 2014. “Structure and Stability of Two Polymorphs of Creatine and Its Monohydrate.” CrystEngComm 16: 8197. https://doi.org/10.1039/C4CE00508B.
Balsom, P. D., K. Söderlund, and B. Ekblom. 1994. “Creatine in Humans with Special Reference to Creatine Supplementation.” Sports Medicine 18 (4): 268–80. https://doi.org/10.2165/00007256-199418040-00005.
Biotechnology Information, National Center for. 2023. “PubChem Compound Summary for CID 586, Creatine.” https://pubchem.ncbi.nlm.nih.gov/compound/Creatine.
Braissant, Olivier, Hugues Henry, Elodie Béard, and Jean Uldry. 2011. “Creatine Deficiency Syndromes and the Importance of Creatine Synthesis in the Brain.” Amino Acids 40 (5): 1315–24. https://doi.org/10.1007/s00726-011-0852-z.
Buford, Thomas W, Richard B Kreider, Jeffrey R Stout, Mike Greenwood, Bill Campbell, Marie Spano, Tim Ziegenfuss, Hector Lopez, Jamie Landis, and Jose Antonio. 2007. “International Society of Sports Nutrition Position Stand: Creatine Supplementation and Exercise.” Journal of the International Society of Sports Nutrition 4: 6. https://doi.org/10.1186/1550-2783-4-6.
Campos-Ferraz, Patricia L, Bruno Gualano, Willian das Neves, Ian V Andrade, Rosa M R Pereira, and Antonio H B Lancha Jr. 2016. “Exploratory Studies of the Potential Anti-Cancer Effects of Creatine.” Amino Acids 48 (8): 1993–2001. https://doi.org/10.1007/s00726-016-2180-9.
Close, Graeme L, David L Hamilton, Andrew Philp, Louise M Burke, and James P Morton. 2016. “New Strategies in Sport Nutrition to Increase Exercise Performance.” Free Radical Biology & Medicine 98: 144–58. https://doi.org/10.1016/j.freeradbiomed.2016.01.016.
Colas, Claire, Lucia Banci, Roberto Martini, and et al. 2020. “Studies of Structural Determinants of Substrate Binding in the Creatine Transporter (CreaT, SLC6A8) Using Molecular Models.” Scientific Reports 10 (1): 6241. https://doi.org/10.1038/s41598-020-63189-z.
Cook, Christian J., Blair T. Crewther, Liam P. Kilduff, Scott Drawer, and Chris M. Gaviglio. 2011. “Skill Execution and Sleep Deprivation: Effects of Acute Caffeine or Creatine Supplementation — a Randomized Placebo-Controlled Trial.” Journal of the International Society of Sports Nutrition 8: 2. https://doi.org/10.1186/1550-2783-8-2.
Dalbo, Vincent J, Michael D Roberts, Jeffrey R Stout, and Chad M Kerksick. 2008. “Putting to Rest the Myth of Creatine Supplementation Leading to Muscle Cramps and Dehydration.” British Journal of Sports Medicine 42 (7): 567–73. https://doi.org/10.1136/bjsm.2007.042473.
Di Biase, Stefano, Xuehui Ma, Xinyi Wang, Jie Yu, Yuchuan C Wang, Derek J Smith, Yan Zhou, et al. 2019. “Creatine Uptake Regulates CD8 t Cell Antitumor Immunity.” The Journal of Experimental Medicine 216 (12): 2869–82. https://doi.org/10.1084/jem.20182044.
Einstein, Albert. 1905. “Zur Elektrodynamik bewegter Körper. (German) [On the Electrodynamics of Moving Bodies].” Annalen Der Physik 322 (10): 891–921. https://doi.org/http://dx.doi.org/10.1002/andp.19053221004.
Feld, Robert D, and David L Witte. 1977. “Presence of Creatine Kinase BB Isoenzyme in Some Patients with Prostatic Carcinoma.” Clinical Chemistry 23 (10): 1930–32.
Gazdar, Adi F, and et al. 1981. “Levels of Creatine Kinase and Its BB Isoenzyme in Lung Cancer Specimens and Cultures.” Cancer Research 41 (7): 2773–77.
Greenwood, Michael, Richard B Kreider, Lori Greenwood, and Allyn Byars. 2003. “Cramping and Injury Incidence in Collegiate Football Players Are Reduced by Creatine Supplementation.” Journal of Athletic Training 38: 216–19.
Groeneveld, Gert Jan, Carola Beijer, Jan H Veldink, Sjon Kalmijn, John HJ Wokke, and Leonard H van den Berg. 2005. “Few Adverse Effects of Long-Term Creatine Supplementation in a Placebo-Controlled Trial.” International Journal of Sports Medicine 26 (4): 307–13. https://doi.org/10.1055/s-2004-817917.
Hultman, Eric, Karin Söderlund, James A. Timmons, Göran Cederblad, and Paul L. Greenhaff. 1996. “Muscle Creatine Loading in Men.” Journal of Applied Physiology (Bethesda, Md. : 1985) 81 (1): 232–37. https://doi.org/10.1152/jappl.1996.81.1.232.
Izquierdo, Mikel, Javier Ibañez, Juan José González-Badillo, and Esteban M. Gorostiaga. 2002. “Effects of Creatine Supplementation on Muscle Power, Endurance, and Sprint Performance.” Medicine and Science in Sports and Exercise 34 (2): 332–43. https://doi.org/10.1097/00005768-200202000-00023.
Joncquel-Chevalier Curt, Marie, Petruta M Voicu, Martine Fontaine, Anne-Frédérique Dessein, Nicole Porchet, Karine Mention-Mulliez, Dries Dobbelaere, Gustavo Soto-Ares, David Cheillan, and Joseph Vamecq. 2015. “Creatine Biosynthesis and Transport in Health and Disease.” Biochimie 119: 146–65. https://doi.org/10.1016/j.biochi.2015.10.022.
Koshy, Kavita M, Edward Giswold, and Edwin E Scheenberger. 1999. “Interstitial Nephritis in a Patient Taking Creatine.” New England Journal of Medicine 340: 814–15. https://doi.org/10.1056/NEJM199903113401017.
Kreider, Richard B., Douglas S. Kalman, Jose Antonio, Tim N. Ziegenfuss, Robert Wildman, Rick Collins,…, and Hector L. Lopez. 2017. “International Society of Sports Nutrition Position Stand: Safety and Efficacy of Creatine Supplementation in Exercise, Sport, and Medicine.” Journal of the International Society of Sports Nutrition 14 (1): 18. https://doi.org/10.1186/s12970-017-0173-z.
Kreider, Richard B, Cate Melton, Christopher J Rasmussen, Mike Greenwood, Sharon Lancaster, Edward C Cantler, Patsy Milnor, and Anthony L Almada. 2003. “Long-Term Creatine Supplementation Does Not Significantly Affect Clinical Markers of Health in Athletes.” Molecular and Cellular Biochemistry 244: 95–104. https://doi.org/10.1023/A:1022469320296.
Kreider, Richard B., and Jeffery R. Stout. 2021. “Creatine in Health and Disease.” Nutrients 13 (2). https://doi.org/10.3390/nu13020447.
Kreider, Richard, Maria Pontes Ferreira, Michael Wilson, and Pamela Grindstaff. 1998. “Effects of Creatine Supplementation on Body Composition, Strength, and Sprint Performance.” Medicine and Science in Sports and Exercise 30 (1): 73–82. https://doi.org/10.1097/00005768-199801000-00011.
Kurth, Isabelle, Naomi Yamaguchi, Carlos Andreu-Agullo, Hong S Tian, Srinivas Sridhar, Shunichi Takeda, Ferdinand C Gonsalves, et al. 2021. “Therapeutic Targeting of SLC6A8 Creatine Transporter Suppresses Colon Cancer Progression and Modulates Human Creatine Levels.” Science Advances 7 (41): eabi7511. https://doi.org/10.1126/sciadv.abi7511.
Lopez, Rebecca M., Douglas J. Casa, Brendon P. McDermott, Matthew S. Ganio, Lawrence E. Armstrong, and Carl M. Maresh. 2009. “Does Creatine Supplementation Hinder Exercise Heat Tolerance or Hydration Status? A Systematic Review With Meta-Analyses.” Journal of Athletic Training 44 (2): 215–23. https://doi.org/10.4085/1062-6050-44.2.215.
Lugaresi, Rafael, Mauricio Leme, Vítor de Salles Painelli, Igor Hideaki Murai, Hamilton Roschel, Marcelo Tatit Sapienza, Antonio Herbert Lancha Junior, and Bruno Gualano. 2013. “Does Long-Term Creatine Supplementation Impair Kidney Function in Resistance-Trained Individuals Consuming a High-Protein Diet?” Journal of the International Society of Sports Nutrition 10 (1): 26. https://doi.org/10.1186/1550-2783-10-26.
Macrae, C. F., I. Sovago, S. J. Cottrell, P. T. A. Galek, P. McCabe, E. Pidcock, M. Platings, et al. 2020. “Mercury 4.0: From Visualization to Analysis, Design and Prediction.” Journal of Applied Crystallography 53: 226–35. https://doi.org/10.1107/S1600576719014092.
Marinello, Poliana C, Paola S Cella, Mônica T J Testa, Paula B Guirro, Wanderson A S Brito, Fernanda H Borges, Rubens Cecchini, Alessandra L Cecchini, José A Duarte, and Rafael Deminice. 2019. “Creatine Supplementation Exacerbates Ethanol-Induced Hepatic Damage in Mice.” Nutrition (Burbank, Los Angeles County, Calif.) 66: 122–30. https://doi.org/10.1016/j.nut.2019.05.004.
Mercimek-Andrews, Saadet, and Gajja S Salomons. 2009. “Creatine Deficiency Disorders.” In GeneReviews®, edited by Margaret P Adam and et al. University of Washington, Seattle.
Miller, E. E., A. E. Evans, and M. Cohn. 1993. “Inhibition of Rate of Tumor Growth by Creatine and Cyclocreatine.” Proceedings of the National Academy of Sciences 90 (8): 3304–8. https://doi.org/10.1073/pnas.90.8.3304.
Ostojic, Sergej M. 2021. “Creatine as a Food Supplement for the General Population.” Journal of Functional Foods 83: 104568. https://doi.org/10.1016/j.jff.2021.104568.
Owen, C. D., P. Lukacik, C. M. Strain-Damerell, A. Douangamath, A. J. Powell, D. Fearon, J. Brandao-Neto, et al. 2023. “SARS-CoV-2 Main Protease with Unliganded Active Site (2019-nCoV, Coronavirus Disease 2019, COVID-19).” Protein Data Bank (PDB).
Papalazarou, Vassilis, Tianzhu Zhang, Nicolle R Paul, Amelie Juin, Marco Cantini, Oliver D K Maddocks, Manuel Salmeron-Sanchez, et al. 2020. “The Creatine–Phosphagen System Is Mechanoresponsive in Pancreatic Adenocarcinoma and Fuels Invasion and Metastasis.” Nature Metabolism 2 (1): 62–80. https://doi.org/10.1038/s42255-019-0159-z.
Passi, Gouri Rao, Swati Pandey, Akella Radha Rama Devi, Ramesh Konanki, Abhishek Ravindra Jain, Shweta Bhatnagar, Ruchi Tripathi, and Vivek Jain. 2022. “Cerebral Creatine Deficiency Disorders – a Clinical, Genetic and Follow up Study from India.” Brain and Development 44 (4): 271–80. https://doi.org/https://doi.org/10.1016/j.braindev.2021.12.004.
Persky, Adam, and Eric Rawson. 2007. “Safety of Creatine Supplementation.” Sub-Cellular Biochemistry 46 (January): 275–89. https://doi.org/10.1007/978-1-4020-6486-9_14.
Poortmans, Jacques R, and Marc Francaux. 1999. “Long-Term Oral Creatine Supplementation Does Not Impair Renal Function in Healthy Athletes.” Medicine and Science in Sports and Exercise 31: 1108–10. https://doi.org/10.1097/00005768-199908000-00005.
Pritchard, Nicola R, and Philip A Kalra. 1998. “Renal Dysfunction Accompanying Oral Creatine Supplements.” The Lancet 351: 1252–53. https://doi.org/10.1016/S0140-6736(05)79319-3.
Qiao, Jia, Ying Su Li, Rong Zeng, Fu Liang Liu, Rui Hua Luo, Chen Huang, Yan Feng Wang, et al. 2021. “SARS-CoV-2 Mpro Inhibitors with Antiviral Activity in a Transgenic Mouse Model.” Science (New York, N.Y.) 371 (6536): 1374–78. https://doi.org/10.1126/science.abf1611.
Trexler, Eric T, Abbie E Smith-Ryan, Erica J Roelofs, Katie R Hirsch, Adam M Persky, and Meredith G Mock. 2016. “Effects of Coffee and Caffeine Anhydrous Intake During Creatine Loading.” Journal of Strength and Conditioning Research 30 (5): 1438–46. https://doi.org/10.1519/JSC.0000000000001223.
Van Cutsem, Jeroen, Bart Roelands, Bert Pluym, Bruno Tassignon, Jo Verschuere, Kevin De Pauw, and Romain Meesun. 2020. “Can Creatine Combat the Mental Fatigue–Associated Decrease in Visuomotor Skills?” Medicine & Science in Sports & Exercise 52 (1): 120–30. https://doi.org/10.1249/MSS.0000000000002122.
Wiroth, Jean-Baptiste, Stéphane Bermon, Sébastien Andreï, Etienne Dalloz, Xavier Hébuterne, and Claude Dolisi. 2001. “Effects of Oral Creatine Supplementation on Maximal Pedalling Performance in Older Adults.” European Journal of Applied Physiology 84 (6): 533–39. https://doi.org/10.1007/s004210000370.
Wu, Guorong, David H. Robertson, Charles L. Brooks, and Michel J. Vieth. 2003. “Detailed Analysis of Grid-Based Molecular Docking: A Case Study of CDOCKER-a CHARMm-Based MD Docking Algorithm.” Journal of Computational Chemistry 24 (13): 1549–62. https://doi.org/10.1002/jcc.10306.
Zhang, Lei, Zehua Zhu, Hailun Yan, Wei Wang, Zhiyong Wu, Fan Zhang, Qianqian Zhang, et al. 2021. “Creatine Promotes Cancer Metastasis Through Activation of Smad2/3.” Cell Metabolism 33 (6): 1111–1123.e4. https://doi.org/10.1016/j.cmet.2021.03.009.